Cite report
IEA (2020), CCUS in Clean Energy Transitions, IEA, Paris https://www.iea.org/reports/ccus-in-clean-energy-transitions, Licence: CC BY 4.0
Report options
Accelerating deployment
Highlights
- The next decade will be critical to the prospects for CCUS and for putting the global energy system on a path to net-zero emissions. A significant scaling-up of CCUS is needed to provide the momentum for further technology development and cost reductions, and to foster progress across a broader range of applications in the longer term.
- Delays in investment and innovation in CCUS technologies would have a lasting impact on future emissions trajectories and could slow the pace at which net-zero emissions can be achieved. A five-year delay in developing and deploying CCUS technologies would halve the CO2 emissions being captured worldwide in 2030 compared to the Sustainable Development Scenario.
- The required rate of CCUS rollout is challenging. It can only be achieved if near-term policy action establishes the conditions for investment along the CCUS value chain. Economic recovery packages are a unique window of opportunity for governments to support CCUS alongside other clean energy technologies.
- The key to successful policy is designing a framework that supports the creation of a sustainable and viable market for CCUS. There is no one-size-fits-all policy template: the appropriate choice or mix of instruments for each country depends on local market conditions and institutional factors. On their own, technology-neutral measures such as carbon pricing are generally not sufficient. Measures targeted at specific CCUS applications, including capital grants and operational support, can help build a business case for investment and drive widespread deployment in the near term.
- There are four high-level priorities for governments and industry to support a rapid scaling-up of CCUS over the next decade: create the conditions to stimulate private investment; target the development of industrial hubs with shared CO2 infrastructure; identify and encourage the development of CO2 storage; and boost innovation to reduce costs and ensure that critical technologies and applications are available, including in sectors where emissions are hard to abate and for carbon removal.
The importance of the next decade
The next decade will be critical to the prospects for CCUS and for putting the global energy system on a path to net-zero emissions. A significant scale-up of deployment is needed to provide the momentum for further technological progress, cost reductions and more widespread application in the longer term. Without a sharp acceleration in CCUS innovation and deployment over the next few years, meeting net-zero emissions targets will be all but impossible.
The introduction of stimulus packages to counter the economic impact of the Covid-19 pandemic presents a unique opportunity for governments to support the needed acceleration in CCUS development and deployment. As previously discussed, CCUS is well placed to contribute to near-term economic recovery plans in some regions, with a growing number of projects that are close to a FID but that will require government support. In particular, industrial applications with highly concentrated CO2 emission streams could be brought online relatively quickly in response to new policy incentives. Support for CO2 storage development as well as innovation is also urgently needed to lay the foundations for future large-scale CCUS deployment.
World CO2 capture capacity additions to 2030 by period and sector in the Sustainable Development Scenario
OpenThe rate of acceleration in CCUS deployment over the next decade that is required for the world to be on track for net-zero emissions is a major challenge. In the Sustainable Development Scenario, the amount of CO2 captured grows by a factor of 20 from around 40 Mt today1 to over 800 Mt in 2030, requiring a significant ramp-up in average annual additions of CO2 capture capacity. The projected rollout is ambitious – as it is for many other emerging low-carbon technologies in the Sustainable Development Scenario – but there are historic precedents for the rapid deployment of similar technologies, notably flue gas desulphurisation.
Most of the growth in CCUS deployment takes place in the second half of the decade. The projected expansion in deployment after 2025 reflects the lead times in developing new CO2 transport and storage infrastructure, which can be as long as ten years, as well as in planning and building capture facilities. Virtually all the capacity that comes online in the next four to five years will already be in advanced development today and/or will have access to existing CO2 transport and storage infrastructure. This highlights the importance of securing a massive increase in investment in the coming months and years for the projected expansion of capacity later in the decade to be achieved.
An analogue to the rapid scaling-up of CO2 capture technology: Flue gas desulphurisation
The near-term growth in CCUS projected in the Sustainable Development Scenario is ambitious, but not without comparable precedent. In the 1970s and 1980s, several countries, including Germany and the United States, introduced regulations to address the problem of acid rain pollution caused in particular by sulphur dioxide emissions from power stations and industrial facilities. These measures led to a rapid expansion of flue gas desulphurisation technology, often known as “scrubbers”. These technologies are comparable to CO2 capture (notably post‑combustion capture) in terms of their complexity, sectors of application and technological characteristics.
Over the period 1972‑2000, an average of 6.6 GW of coal-fired power generation capacity was equipped with scrubbers each year globally. In Germany alone, the rate of deployment reached an annual average of 9 GW from 1985‑88 (IEAGHG, 2012). In China, the installation of scrubbers accelerated in the 2000s, contributing significantly to overall global deployment of the technology. At its peak (around 2002‑12), an annual average of 73 GW of capacity was equipped with scrubbers worldwide (van Ewijk and McDowall, 2020). In the Sustainable Development Scenario, CO2 capture facilities come online at much lower rates in the power sector, averaging 23 GW/year over the entire projection horizon. Deployment peaks at around 40 GW/year in the 2060s, driven mainly by increasing capture from bioenergy power stations – 45% less than the peak in scrubbers and 90% less adjusted for the size of the economy.
This suggests that the increase in CO2 capture equipment projected in the Sustainable Development Scenario over the next decade and beyond is technologically achievable – if effective policy or regulatory frameworks are in place and if transport and storage infrastructure are scaled at a similar rate. In practice, a significant and sustained boost in innovation to demonstrate and commercialise CO2 capture in heavy industry, in addition to significant public support, will also be needed.
The projected growth in CO2 capture over the next decade in the Sustainable Development Scenario is characterised by three important trends:
- Retrofitting of large numbers of existing power and industrial facilities that significantly reduces lock-in of emissions. An annual average of around 20 coal power plants are retrofitted with capture equipment between 2025 and 2030, primarily in Asia.2 In 2030, over 450 Mt of CO2 is captured from retrofitted fossil fuel-based power and industrial facilities, of which the majority is permanently stored. These retrofits result in the avoidance of over 70 Gt of cumulative emissions over the lifetime of the plants – more than double the level of global annual energy-sector emissions today.3
- The scale-up of low-carbon hydrogen production with CCUS. With a relatively concentrated CO2 stream, hydrogen represents a lower-cost opportunity for CCUS deployment compared with power generation, for example. By 2030, 18 Mt of hydrogen is produced from CCUS-equipped facilities in the Sustainable Development Scenario.
- The rapid adoption of CCUS technologies and applications that are not yet widely used. Around half of the CO2 captured in the period to 2030 is from technologies and applications that are currently in the prototype or demonstration phase, including capture technologies in heavy industry where alternative low-carbon technologies are more costly or difficult to adopt. Between 2025 and 2030, an average of 90 cement plants are equipped with CCUS each year.4 DAC also starts to be deployed commercially, primarily for synthetic fuel production, with capacity growing from around 9 000 t today to 10 Mt in 2030.
Delays in investment and innovation in CCUS technologies would have a lasting impact on future emissions trajectories and affect the pace at which net-zero emissions can be achieved. The IEA calculates that a five-year delay in completing demonstration projects for pre‑commercial CCUS technologies, together with a slowdown in the deployment of CCUS technologies at early adoption stage, would result in 50% less CO2 emissions being captured worldwide in 2030 and 35% less in 2040 than in the Sustainable Development Scenario (IEA, 2020). CO2 captured from cement production and power generation would be the areas most affected, accounting for almost 80% of the reduction in CCUS deployment through to 2040. The delay in deploying CO2 capture technologies would also hold up the rate of decline in costs over time, due to the missed opportunity for learning-by-doing.
Policy considerations
Supporting accelerated deployment
The rapid deployment hinges critically on a massive increase in government support, as well as new approaches to public and private investment. CCUS is not unique in this respect: the future of many of the clean energy technologies needed in the global energy transition depends on rigorous and sustained policy action.
CCUS faces some specific challenges in the initial scaling-up phase, which policies must recognise and address. These include the need for co‑ordination across multiple sectors and stakeholders; high capital investment requirements for CO2 capture and related infrastructure; uncertainty surrounding long-term ownership and liability for stored CO2; untested insurance and finance markets; and public opposition to storage (particularly onshore) in some regions.
The key to successful policy is designing a framework that supports the creation of a sustainable and viable market for CCUS. That framework needs to recognise that the private sector is unlikely to invest in the technology unless it is obliged to do so, or unless it can make a profit from the sale of the CO2 or by earning credits from the emissions avoided under carbon pricing arrangements.
A range of policy instruments are at policy makers’ disposal to support the establishment of a market for CCUS and address the investment challenges. In practice, a mix of measures is likely to be needed. These measures include direct capital grants, tax credits, carbon pricing mechanisms, operational subsidies, regulatory requirements and public procurement of low-carbon products from CCUS-equipped plants. Continuous support for innovation is also needed to drive down costs, and develop and commercialise new technologies.
Main policy instruments for CCUS development and deployment
Category |
Types |
Examples |
---|---|---|
Grant support |
Capital funding provided directly to targeted projects or through competitive programmes to overcome high upfront costs. |
|
Operational subsidies |
|
|
Carbon pricing |
|
|
Demand-side measures |
|
|
CCUS-specific market mechanisms |
|
|
Regulatory standards and obligations |
|
|
Risk mitigation measures |
|
|
Innovation and RD&D |
|
|
Almost all CCUS projects operating today have benefited from some form of public support, largely in the form of capital grants and – to a lesser extent – operational subsidies. Grant funding has played a particularly important role in projects coming online since 2010, with 8 out of 15 projects receiving grants ranging from around USD 55 million (AUD 60 million) in the case of Gorgon in Australia to USD 840 billion (CAD 865 million) for Quest in Canada. Seven projects have had access to operational support in the form of tax credits or subsidies, including US projects developed since 2009, which can access the original5 45Q tax credit of USD 20/tCO2 for geological storage and USD 10/tCO2 used in EOR. Of the seven projects that that have not received a direct capital subsidy, four are owned and operated by a state-owned enterprise.
Policy support for large-scale CCUS projects in operation today
Policy/support measure: |
|||||||
---|---|---|---|---|---|---|---|
Country |
Project (Operational date) |
Sector |
Carbon tax |
Grant support |
Operational support |
Regulatory requirement |
State-owned enterprise |
US |
Terrell natural gas plants (1972) |
Fuel transformation |
|
|
|
|
|
US |
Enid Fertilizer (1982) |
Industry (chemicals) |
|
|
|
|
|
US |
Shute Creek gas processing (1986) |
Fuel transformation |
|
|
|
⬤ |
|
Norway |
Sleipner CO2 storage (1996) |
Fuel transformation |
⬤ |
|
|
|
⬤ |
US/ |
Great Plains Synfuels (2000) |
Fuel transformation |
|
|
|
|
|
Norway |
Snøhvit CO2 storage (2008) |
Fuel transformation |
⬤ |
|
|
|
⬤ |
US |
Century Plant (2010) |
Fuel transformation |
|
|
⬤ |
|
|
US |
Air Products steam methane reformer (2013) |
Fuel transformation |
|
⬤ |
⬤ |
|
|
US |
Lost Cabin Gas Plant (2013) |
Fuel transformation |
|
|
⬤ |
|
|
US |
Coffeyville Gasification (2013) |
Industry (chemicals) |
|
|
⬤ |
|
|
Brazil |
Petrobras Santos Basin pre‑Salt oilfield (2013) |
Fuel transformation |
|
|
|
|
⬤ |
Canada |
Boundary Dam (2014) |
Power generation |
|
⬤ |
|
⬤ |
⬤ |
Saudi Arabia |
Uthmaniyah CO2-EOR (2015) |
Fuel transformation |
|
|
|
|
⬤ |
Canada |
Quest (2015) |
Fuel transformation |
|
⬤ |
⬤ |
|
|
UAE |
Abu Dhabi (2016) |
Industry (steel) |
|
|
|
|
⬤ |
US |
Petra Nova (2017) |
Power generation |
|
⬤ |
⬤ |
|
|
US |
Illinois Industrial (2017) |
Fuel transformation |
|
⬤ |
⬤ |
|
|
China |
Jilin oilfield CO2-EOR (2018) |
Fuel transformation |
|
|
|
|
⬤ |
Australia |
Gorgon CO2 Injection (2019) |
Fuel transformation |
|
⬤ |
|
⬤ |
|
Canada |
ACTL – Agrium (2020) |
Industry (Chemicals) |
|
⬤ |
|
|
|
Canada |
ACTL – North West Sturgeon Refinery (2020) |
Fuel transformation |
|
⬤ |
|
|
|
Source: Global CCS institute (GCCSI) (2019), The Global Status of CCS 2019.
An explicit carbon price or tax has supported CCUS investment in only two cases to date: the Sleipner and Snøhvit projects in Norway, which were subject to a CO2 tax on offshore oil and gas production introduced in 1991. In both cases, there was a technical need to separate the CO2 from the natural gas to meet market requirements, strong subsurface expertise and knowledge within Equinor,6 favourable geology, relatively high product margins, and a lack of alternative abatement options.
There is no one-size-fits-all policy for CCUS
There is no one-size-fits-all policy template for CCUS. The appropriate choice or mix of instruments for each country depends on local market conditions and institutional factors, including the current stage of CCUS infrastructure development, emissions targets, domestic energy resources, and the availability and cost of alternative approaches to cutting emissions. These factors vary markedly across regions:
- In the United States, CCUS has been supported through grant funding and an extensive R&D programme. The expanded 45Q tax credits are proving effective in stimulating plans for new projects. Tax credits are a familiar and well-established policy mechanism in the country, having been extensively used to support the development of renewable energy technology. They may not be as effective in other regions where CCUS is at an earlier stage of development or if higher-cost industrial applications are being targeted.
- Europe has only two operational CCUS projects but a rich pipeline of planned projects concentrated around industrial clusters with shared CO2 storage infrastructure and applications that include cement, gas-fired power generation, waste-to-energy and hydrogen production (see Chapter 4). A combination of competitive grant funding (for example, through the EUR 10 billion Innovation Fund and the GBP 800 million UK CCS Infrastructure Fund), direct government funding with risk-sharing arrangements (Norway’s Longship CCS project) and operational support (for example the Dutch SDE++ scheme) are the main measures currently in place to support the expansion of CCUS.
- In countries or regions with large state-owned enterprises, including China and the Middle East, direct investment has been used to support early CCUS projects. State-owned enterprises could lead the more widespread application of CCUS and in some cases help to create a low-carbon market through procurement policies.
The suitability of each type of policy instrument also varies according to the specific application of CCUS. Some capture applications, such as natural gas processing, are mature and relatively less expensive, requiring relatively limited policy intervention. Other applications, including in heavy industry, are currently at an earlier stage of development, and the higher costs of CO2 capture and its potential impact on competitiveness – particularly in the case of internationally traded commodities, such as steel – are a major barrier to the adoption of CCUS. Transport and storage infrastructure is capital-intensive and future demand from capture facilities can be uncertain, creating considerable risk for project developers and investors. Government support and co‑ordination will be very important in developing new transport and storage infrastructure as CCUS scales up.
Policy implications of CCUS deployment by sector
|
Sector characteristics |
Implications for policy |
---|---|---|
Transport and storage |
Likely monopolistic market for CO2 transport |
Transport and storage assets may need to be regulated or owned/operated by governments |
High capital costs of transport and storage infrastructure |
Grant funding or financing support may be needed |
|
Co‑ordination and alignment of timelines with capture development needed |
Targeted funding of integrated projects (e.g. around industrial hubs) or volume guarantees |
|
Uncertainties over long-term ownership and liability for stored CO2 |
May require public liability cap or transfer of ownership to governments post‑closure |
|
Industry |
International competiveness for traded commodities with low profit margins |
Compensate for additional cost; mitigate additional cost along the value chain; consider border adjustments; create demand for low-carbon products |
Range of applications, technologies and scale |
Policies and implementation models must be flexible |
|
Current high cost of capture in many applications |
RD&D required |
|
High credit risk, uncertain markets and expected short payback |
Capital financing and operational support may be required |
|
Hydrogen production |
Low cost of CO2 capture |
Smaller financial incentive for CO2 capture than in other sectors may be needed |
Uncertainty over future demand |
Policy support to stimulate demand for low-carbon hydrogen in heat, industry and/or transport |
|
End use may be in clusters of high energy demand and/or production |
Focus on early opportunities by incentivising production in industrial clusters with CCUS infrastructure |
|
Power generation |
High capital cost of retrofits |
Capital support or guaranteed operational revenue |
Power markets vary significantly among regions and countries |
Different policies required nationally; experience in other regions not always transferable |
|
Load factors for plants equipped with CCUS may fall over time as more renewables are integrated |
Flexibility to accommodate a potential transition from baseload to flexible generation |
|
CO2 use |
Uncertain climate benefits
|
Policy incentives need to be linked to robust life-cycle assessments of emissions savings |
Uncertain demand for CO2 use |
Public procurement measures to consider CO2-derived products |
|
High energy needs and costs for CO2 conversion to fuels and chemicals |
RD&D support to reduce costs and prove feasibility of technology at scale |
|
Carbon removal |
Immature application with uncertain costs and demand |
Focus on proving feasibility at scale and lowering costs; stimulate demand through targets or CO2 certificate trading |
No recognition of value of negative emissions |
Include carbon removal in emissions trading frameworks or other tradeable credits |
|
Measurement and verification of carbon removal |
Policy incentives need to be linked to robust life-cycle assessments of emissions savings |
Policy action also needs to be tailored to the stage of CCUS technology development in the sector or application in which it is being deployed. As deployment increases, the market for CCUS should become progressively more independent, requiring less government intervention. Targeted subsidies could be phased out and economy-wide measures such as carbon pricing could become the primary measure to support investment.
Today, CCUS is at an early stage of commercialisation, with most applications classified as demonstration and early adoption in this report. On their own, technology-neutral measures such as carbon pricing are generally not sufficient to overcome the barriers to commercialising these or other emerging technologies. Measures targeted at specific CCUS applications, including (initially) capital grants and operating subsidies, would likely be required to build a business case for early investment and to drive widespread deployment.
CCUS Policy Measures By Stage Of Technology Development
Open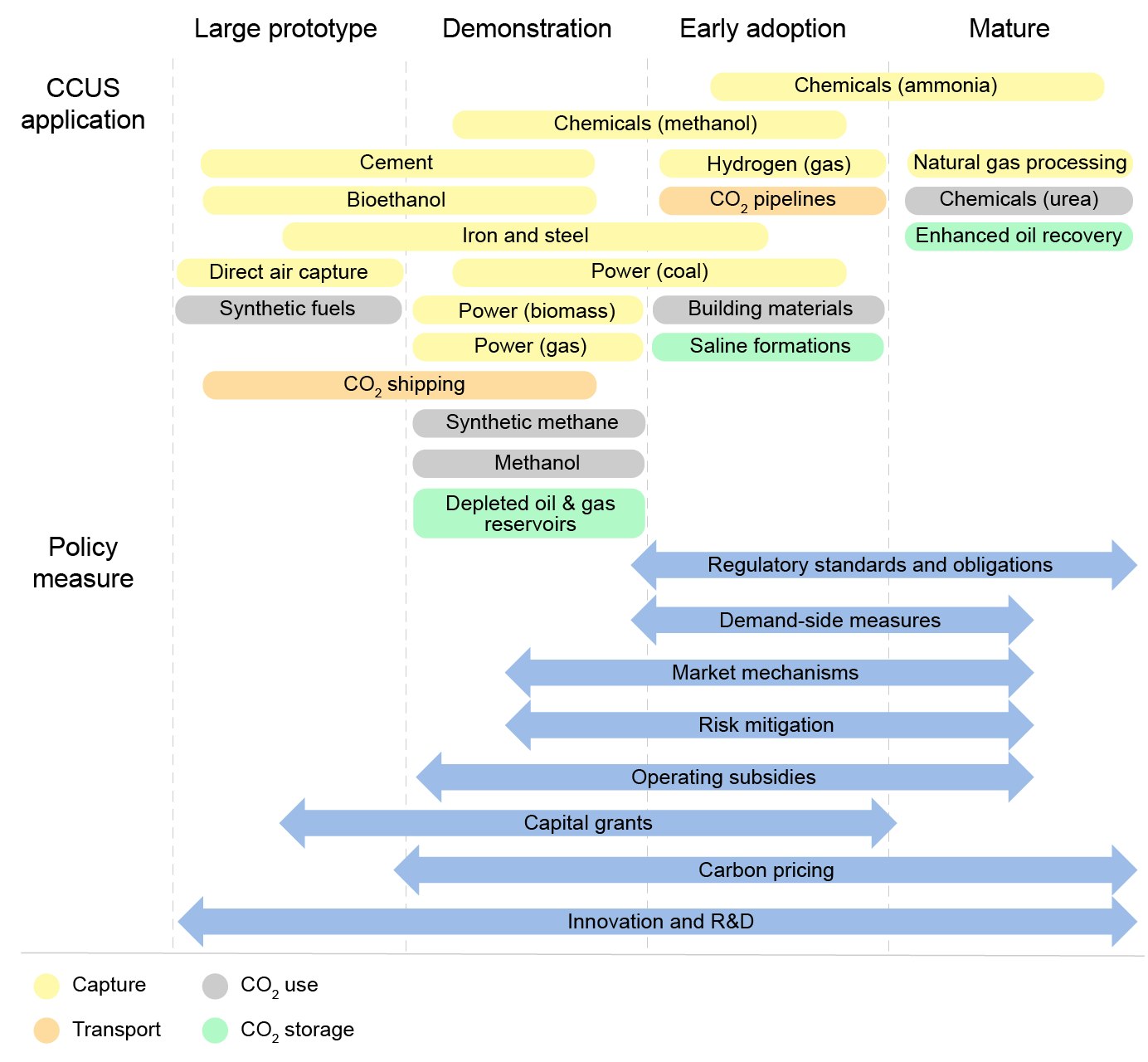
Priorities for accelerated deployment
Recognising that policy responses will need to be tailored to national and sector-specific circumstances, there are four high-level priorities for governments to support a rapid scaling-up of CCUS over the next decade:
- Create the conditions to stimulate private investment.
- Target the development of industrial hubs with shared CO2 infrastructure.
- Identify and encourage the development of CO2 storage.
- Boost innovation to reduce costs and ensure that critical technologies are available this decade.
Create the conditions for investment
Bringing forth the investment needed to deploy CCUS on the scale envisioned in the Sustainable Development Scenario requires measures to make such investment commercially attractive. An estimated USD 160 billion of cumulative investment in CCUS is needed to 2030 in that scenario, a tenfold increase from the decade to 2020. While a range of specific policy measures could be employed to support this investment depending on national circumstances or preferences, their overall effectiveness would be improved by three key approaches: placing a value on emissions reductions, provide funding to support capital and operating costs, and allocating risks between the public and private sector.
Place a value on reducing emissions
A major barrier to CCUS deployment today in many regions is the low value or absence of a value attached to CO2 emissions reductions, such that there may be no commercial driver for industrial or power facilities to capture CO2 as an alternative to emitting it, even where this can be done at relatively low cost. Placing a value on reducing emissions does not necessarily require explicit carbon pricing through emissions trading or explicit taxation of emissions; though these can play an important role. It can also take the form of public procurement programmes that favour lower-emissions commodities or products, or tax credits associated with CO2 storage.
Provide funding to support capital and operating costs for early projects
Grant funding programmes can play an important role in supporting early CCUS deployment, particularly first-of-a-kind projects and CO2 transport and storage infrastructure. They can alleviate the high capital costs and commercial and technical risks associated with such projects. However, they can be a heavy burden on public budgets, limiting their use to a small number of individual facilities. A rapid scale-up of CCUS will necessitate a shift towards market-based measures that can complement grant funding and provide a stable and ongoing framework for CCUS facilities to operate over the long term.
Allocate risks across the public and private sector
The private sector can manage the majority of risks associated with CCUS projects, but some may need to be shared initially with governments. These include low-probability but high-impact risks, such as the long-term liability associated with CO2 storage, including the risk that the CO2 could migrate or leak out many years or decades after the site has closed. This risk is difficult to quantify and hard to insure against, in part because the financial cost to the project would depend on the prevailing carbon price at the time of the leakage. Policy or legislative frameworks should consider the option to transfer the ownership of the stored CO2 back to governments after a specified period and with appropriate assurances, including evidence that the injected CO2 behaving in a stable and predictable manner. Other risks that can inhibit investment include cross-chain risk – that a failure of one of the components in the CCUS supply chain affects operations in other parts of the chain – and stranded assets, such as when storage capacity is built but no CO2 is available due to a lack of investment in capture facilities. Governments can play a critical role in co‑ordinating and orchestrating CCUS deployment in its early stages to alleviate these risks (e.g. the Norwegian government with its longship CCS project).
Target industrial hubs with shared CO2 infrastructure
The development of CCUS hubs with shared CO2 transport and storage infrastructure could play a critical role in accelerating the scale-up of CCUS by exploiting economies of scale and making it feasible to capture CO2 at smaller industrial facilities, for which dedicated CO2 transport and storage infrastructure may be both impractical and uneconomic. It can allow continued operation of existing infrastructure and supply chains in industrial regions, retaining employment and boosting the potential to attract new investment, including in energy-intensive industries or low-carbon hydrogen production.
To drive the development of hubs, governments first need to identify opportunities in partnership with potential investors and then establish business models that encourage their development.
Identify opportunities for CCUS deployment in industrial regions
A national or regional audit of the emissions associated with industrial clusters – including the age and type of facilities – together with an assessment of CO2 storage options and opportunities for using the CO2 is needed to inform the planning and development of infrastructure. Early planning and co‑ordination can promote more efficient investment decisions in the long term, including the identification of opportunities for economies of scale through “oversizing” of infrastructure to accommodate future demand. Governments can play a leading role in this planning and co‑ordination across regions and industries (e.g. the Alberta Carbon Trunk Line in Canada).
Establish a business model for CO2 transport and storage infrastructure
Like electricity and natural gas networks, CO2 infrastructure may be considered a natural monopoly, whereby high start-up costs and economies of scale make it economically more efficient to have a single owner and operator rather than competing providers. A number of business models have been proposed for the development of industrial CCUS hubs (in CCUS Cost Challenge Taskforce, 2018; IEAGHG, 2018; Element Energy 2018). Virtually all involve the CO2 transport and storage infrastructure being owned and operated by a dedicated entity that is publicly owned (wholly or partly) or regulated. An exception involves hubs built around an “anchor” capture facility (often a power plant) providing sufficient scale for the initial transport and storage infrastructure development, with third-party access being granted to neighbouring CO2 capture facilities.
Identify and encourage the development of CO2 storage
Confidence in the availability of safe, secure and adequate CO2 storage is a prerequisite for investment in both transport and storage infrastructure and capture facilities. Global CO2 storage resources are considered to be well in excess of likely future requirements. In many regions, however, significant further assessment work is required to convert theoretical storage capacity into “bankable” storage, whereby the maximum amount of CO2 that can ultimately be stored, the maximum rate of injection, how the gas is contained in the formation and the risk of leakage are well understood. The identification and development of CO2 storage will also need to be supported by a robust legal and regulatory framework, as well as effective communication with local communities and the broader public.
Develop a geological CO2 storage atlas
The process of characterising and assessing CO2 storage can be lengthy – up to ten years depending on existing data (see Chapter 3). This underscores the need for early action. Government geological surveys should start by undertaking pre‑commercial CO2 storage assessments in order to develop an atlas of CO2 storage resources (as with other natural resources, the knowledge obtained can be considered a public good). Oil and gas companies will be important partners in this work in countries where they have been active, as they will already hold large amounts of data, notably on depleted oil and gas reservoirs. Storage atlases have already been developed in several regions, and the OGCI and Global CCS Institute recently released a global CO2 storage resource database that covers 13 countries and regions (OGCI, 2020). Beyond the pre‑competitive assessment stage, policy measures will be needed to support investment in the detailed characterisation and assessment of storage sites.
Establish a legal and regulatory framework for safe and secure storage
The development of CO2 storage resources must be underpinned by a robust legal and regulatory framework. This should ensure appropriate site selection and safe operation of CO2 storage, providing a framework to mitigate and manage risks across all stages of site development, operation and closure. It should also provide a legal basis for CO2 storage, allocating property rights, managing competition for resources (for example, with oil and gas development), and defining roles and responsibilities, including ownership and liability for stored CO2. International standards that have already been developed for CO2 storage (e.g. ISO/TC 265, ISO 27914) can inform national efforts to establish such a framework.
Support public awareness and education
Successful deployment of CCUS will involve a concerted effort to ensure that local communities and the general public understand and accept the technology. Geological storage of CO2 can raise legitimate concerns about safety and risks, since it is a relatively new concept for many people. Communication and engagement should be initiated at the earliest possible stage of project development to secure community support – as was the case for Shell’s Quest project in Canada and the Tomakomai project in Japan. Governments, alongside non‑governmental organisations and the scientific community, will also have an important role to play in communicating the value of CCUS in the portfolio of technologies needed to meet climate goals.
Boost technology innovation
Innovation will be key to scaling up CCUS in both the short and long term. In the Sustainable Development Scenario, around 60% of the cumulative emissions reductions through to 2070 hinge on technologies that are currently at the prototype or demonstration phase, including carbon removal (BECCS and DAC), new ways of using CO2 (particularly to make fuels and chemicals), and CO2 capture in cement and iron and steel production. Governments and industry can drive their deployment through accelerated innovation, collaborative RD&D and direct support for emerging technologies.
Private-sector entrepreneurs, companies and financiers are expected to play a critical role for innovation in clean energy technologies such as CCUS. Private-sector participants in the innovation system greatly outnumber those from the public sector, with public-sector employees representing just 5-25% of R&D researchers in most member countries of the Organisation for Economic Co‑operation and Development (OECD) (OECD, 2020). Success will depend upon the public and private sectors working closely together to agree on the way ahead, identify projects and metrics, and learn together from past successes and failures.
Reduce CO2 capture costs and support new technologies through RD&D
CO2 capture typically accounts for almost 75% of the cost of CCUS and can range from USD 15-25/t to more than USD 120/t, depending on the application and the concentration of CO2. Although capture costs have already declined substantially in the past decade, RD&D will play a critical role in supporting further cost reductions.
RD&D efforts should focus on developing and demonstrating the technical performance and costs of each element of the CCUS value chain (capture, transport, use and storage), as well as their successful integration. Technological development is critical to prepare various CCUS applications – ranging from cement and iron and steelmaking to synthetic fuels and DAC – for large-scale deployment. RD&D programs should aim to further improve mature capture technologies, in particular chemical absorption through better solvent design and automated operating systems to optimise capture processes, but also technologies and applications that are still at earlier stages of development. Focus RD&D areas for these novel applications include designing better separation materials, lowering energy consumption, and integration with the core process. Transport and storage technologies would benefit from developments in digitalisation, including advanced modelling, sensing and real-time monitoring technologies to accelerate site appraisals and improve tracking of CO2.
Accelerate the availability of CCUS in key applications
Several CCUS technologies and applications will need to rapidly progress from the prototype or demonstration stage to being commercially available within the next decade. There is an urgent need to scale-up key applications, particularly in heavy industry (cement, steel and chemicals), CO2 use (for synthetic fuels) and for carbon removal, where the technologies are generally at an earlier stage of development but where CCUS plays a critical role in the transition to net zero.
Targeted and tailored support for innovation in these applications will be needed, and could also be a focus for international collaboration. Where budgets allow, governments should increase funding for these technologies given their long-term strategic importance and the need to improve understanding of their technical potential and cost. For industrial applications, including cement and steel production, the availability of shared CO2 transport and storage infrastructure will be important to improve the economics of CO2 capture given the relatively smaller quantities of CO2.
Collaborate to support CCUS innovation
Risks from being a first mover can sometimes be too high for a single country to fund if the market players are multinational, the outlook uncertain and the project requires significant upfront capital – as is currently the case for CCUS, including for low-carbon hydrogen and industrial processes. Countries with smaller R&D budgets and companies with weaker balance sheets are likely to find collaboration especially attractive. International partnerships and initiatives, such as the Clean Energy Ministerial CCUS Initiative, Mission Innovation, IEA Greenhouse Gas Technologies Programme (IEAGHG) and the Accelerating CCUS Technologies (ACT) programme are providing an important foundation for collaboration on CCUS development and deployment.
References
Includes CO2 captured from large-scale CCUS facilities where the CO2 is stored or used for EOR (see Chapter 1) but not CO2 that is generated in ammonia production and used on-site to manufacture fertiliser (as this use of CO2 is not associated with a climate benefit).
Based on coal-fired power plants with an average CO2 capture capacity of 2 Mt.
Assuming a typical operating life for coal-fired power plant of 50 years.
Based on cement plants with annual average CO2 emissions of 0.5.
The 45Q tax credit was expanded and increased in 2018, to reach USD 50/tCO2 for geological storage and USD 35/tCO2 for CO2 used in EOR (or for other beneficial uses). The original tax credit was capped at 75 000 tCO2, cumulative, and it is not clear if all eligible US projects have accessed this. The cap was removed in 2018.
Formerly Statoil, the state oil company and project operator.
Reference 1
Includes CO2 captured from large-scale CCUS facilities where the CO2 is stored or used for EOR (see Chapter 1) but not CO2 that is generated in ammonia production and used on-site to manufacture fertiliser (as this use of CO2 is not associated with a climate benefit).
Reference 2
Based on coal-fired power plants with an average CO2 capture capacity of 2 Mt.
Reference 3
Assuming a typical operating life for coal-fired power plant of 50 years.
Reference 4
Based on cement plants with annual average CO2 emissions of 0.5.
Reference 5
The 45Q tax credit was expanded and increased in 2018, to reach USD 50/tCO2 for geological storage and USD 35/tCO2 for CO2 used in EOR (or for other beneficial uses). The original tax credit was capped at 75 000 tCO2, cumulative, and it is not clear if all eligible US projects have accessed this. The cap was removed in 2018.
Reference 6
Formerly Statoil, the state oil company and project operator.