IEA (2020), Introduction to System Integration of Renewables, IEA, Paris https://www.iea.org/reports/introduction-to-system-integration-of-renewables, Licence: CC BY 4.0
Technology options
Different technology options can provide system flexibility across the various timeframes.
Power plants
Conventional power plants are currently the predominant source of system flexibility in modern power systems. Flexible power plant operation can take many forms, from rapidly changing plant output, to starting and stopping more quickly, to turning down plant output without triggering a shutdown. There are two main categories of strategies that can make existing conventional power plants more flexible:
- Changes to operational practices require lower capital investment levels and can be enabled by improved data collection and real-time monitoring.
- Flexibility retrofit investments may require higher investment levels but may enable existing assets to meet new flexibility requirements, for example by coupling with a battery energy storage system.
Southern California Edison’s Center Peaker plant in Norwalk, California provides a key example of an innovative flexibility retrofit investment of an existing power plant. In this case, a natural gas peaking power plant was coupled to a 10 MW/ 4.3 MWh battery, enabling the plant to offer spinning reserves without burning any fuel, while also offering valuable frequency response services. The battery storage component of the hybridised power plant covers the spinning reserve requirements during the first few minutes required for the gas plant to start-up, after which the plant can ramp up to full capacity while the battery output decreases.
Variable renewable energy
Variable renewable energy (VRE) is often perceived as the key driver of new flexibility requirements, yet VRE power plants can also provide flexibility services. However enabling the utilisation of their full range of technical capabilities requires adequate technical requirements, and in some cases economic incentives.
First, it is necessary to have appropriate connection codes that specifically require VRE to provide flexibility services. Due to their highly technical nature, connection codes rarely receive adequate policy attention, but amending them can help to increase the visibility and controllability of VRE resources to system operators.
Second, VRE resources are commonly remunerated on a volumetric basis for the energy they produce. Yet in some cases they also provide flexibility services that require reductions in energy production. This means that it may be necessary to ensure that VRE generators are remunerated fairly for providing flexibility services, similar to conventional power plants.
Generally, advanced VRE resources can be operated flexibly by either running at full output and dispatching downward when needed, known as downward dispatch, or running at a reduced output and using that extra capacity headroom to be dispatched upward or downward when needed, known as full flexibility.
A study by Energy and Environmental Economics examined the impact of these operating modes in the Tampa Electric Company (TECO) system in Florida, showing that as annual solar PV penetration increases on the grid, flexible operation of solar PV resources provides greater operational cost savings than must-run operation.
As TECO is an integrated utility, it is able to benefit from avoided fuel costs and has an incentive to run its PV assets flexibly. In restructured markets, the adoption of flexible VRE operation modes may require opening up new revenue streams to balance foregone revenues from otherwise fixed feed-in tariffs. This can be done either through increasing market risk through direct marketing as in Germany, introducing sliding feed-in premiums or opening up ancillary services markets.
Modelled annual operational cost savings for the TECO power system in Florida, as a percentage of total system operational costs without solar PV generation
OpenDistributed energy resources
Distributed energy resources (DER) refers to a broad category on resources including: distributed generation, distributed battery storage, demand response and electric vehicles. DER have a number of benefits for individual customers and also have the potential to be aggregated and leveraged to provide system flexibility services at the local and bulk power system levels. However, taking advantage of these opportunities may require various changes to connection codes, regulation and market rules.
Up until recently, much of DER participation in demand-side management programmes has been driven by peak demand reduction. However looking forward, DER will increasingly help to meet additional system flexibility needs.
For example in Germany, the SINTEG project has enabled a number of so-called “regulatory sandboxes” to test the feasibility of new flexibility solutions in specific regions. The Enera project in northwestern Germany is being tested in a region where local VRE generation is equivalent to 235% of local annual demand. The project aims to avoid short-term distribution network congestion and redispatch costs by piloting a local flexibility services platform for market-based congestion management.
Storage
Electricity storage describes all technologies that can absorb electrical energy and then later return it as electrical energy. Arbitrage opportunities have driven storage deployment in the last 40 years, in particular pumped storage hydro.
Electricity storage technologies can provide multiple flexibility services ranging the ultra-short- to the long-term, helping to accommodate increasing VRE shares. Recently, battery storage technologies have seen rapid cost declines, and although they are not suitable for seasonal storage, they could play a greater flexibility role in the short-term timescale in future power systems. Another promising future development is the emergence of large grid-scale storage based on second-use batteries.
One of the key advantages of storage (and particularly battery energy storage) is its technical capability to offer multiple sources of value for the power system. In this context, storage is often discussed as having the ability to “stack value”. However, in many contexts, policy, market and regulatory frameworks do not allow technically capable resources to provide multiple services simultaneously. Allowing for value stacking can help realize the full value of battery storage but it is key to ensure that service reliability remains a priority.
One notable example is the 100MW/129MWh Hornsdale Power Reserve in Australia – one of the world’s largest lithium-ion batteries – which participates in regulation, contingency reserve and energy markets in the Australian National Electricity Market. Built with support from the State Government of South Australia to improve power system security, the battery has multiple value streams. This is possible through multiple overlaying contracts attached to particular capacity blocks of the battery. The battery’s deployment has contributed to reducing the price of ancillary services while improving system reliability.
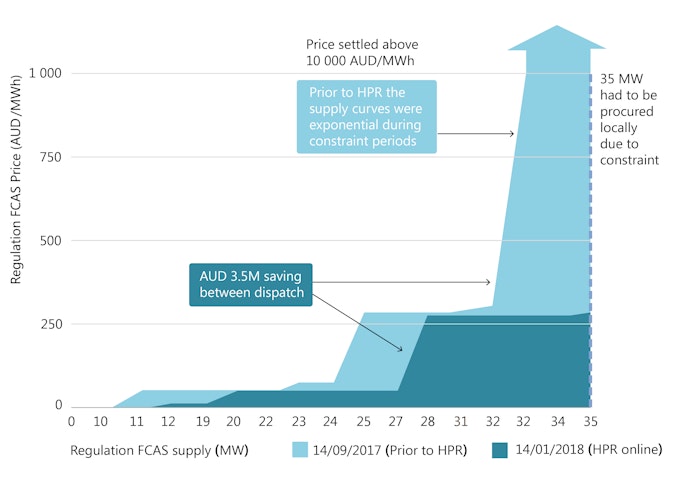
Source: Aurecon (2018), Hornsdale Power Reserve: Year 1 Technical and Market Impact Case Study.
Electricity network assets
The benefits of electricity networks and interconnections (intra- and inter-regional) cut across all aspects of the power sector, including: (a) improved security of supply; (b) improved system efficiency; and (c) improved integration of VRE resources. Electricity networks enable system flexibility by allowing a broader set of flexible hardware resources to be shared across different geographical regions. Today, significant flexibility resources are still being underutilised due to transmission and interconnection bottlenecks. Policy makers have a critical role to play in supporting grid interconnectivity which enables power system flexibility.