Heating and Cooling Strategies in the Clean Energy Transition
Outlooks and lessons from Canada’s provinces and territories
About this report
Introduction
The buildings sector represents a major share of Canada’s energy demand, accounting for about a quarter of total final energy consumption. New building construction continues to be a strong driver of demand for energy services in buildings, with floor area in Canada having increased about 5% faster than population did in the last decade. Canada’s climate also strongly influences energy use for needs such as heating and cooling, which together represent around 65% of the sector’s energy consumption.
Progress over the past two decades, including effective implementation of Canada’s National Building Code and related provincial standards, has dampened the impact of a growing buildings sector. End-use equipment in buildings is also increasingly more efficient, as can be seen from Canada’s shift to more efficient condensing furnaces. The result is that energy consumption in the buildings sector has remained relatively stable since 2007, although winter conditions and subsequent heating demand still cause fluctuations in buildings sector energy use.
Looking forward, expansion of Canada’s efforts will require greater emphasis on energy intensity improvements of existing buildings, which are expected to account for around half of Canada’s total buildings stock in 2050. Strategies around heating and cooling demand will play a major role in achieving ambitions for buildings in the clean energy transition, but they will depend on a multitude of factors, including local climate, energy prices, building type and possible technology trade-offs – for instance, using high-performance heating equipment with less building envelope improvement.
Building energy demand can be cut without reducing quality of services
In the Clean Technology Scenario (CTS), energy demand in Canada’s buildings sector falls more than 35% by 2050 relative to 2018, even with floor area expected to grow by as much as 40% and without reducing the level of energy services in buildings. About 85% of those reductions come from heating and cooling, without reducing energy services in Canada’s growing buildings sector. This is starkly different from expected trends in the Reference Technology Scenario (RTS), in which energy use only begins to decline slowly in the 2040s.
Energy demand in Canada’s buildings could decrease by as much as 35% by 2050 relative to 2018 using known technology solutions and without reducing building energy services
Buildings energy demand in Canada in the CTS
The decline in energy demand, including in particular a steady drop in fossil fuel use, means buildings-related carbon dioxide (CO2) emissions fall dramatically in the CTS, using energy technology solutions already known today. High-performance new construction such as near-zero energy buildings (nZEBs) and deep energy renovation of existing buildings play a key role in achieving those energy savings and emissions reduction, as do energy-efficient and low-carbon technologies such as electric, gas thermal and hybrid heat pumps. Simultaneous reductions in power sector CO2 intensities mean the overall carbon footprint of the buildings sector by 2050 is under 25 million tonnes of CO2 (MtCO2), around 20% of current levels.
Buildings-related CO2 emissions are 80% lower than in 2018 by 2050 in the CTS, thanks to major energy intensity improvements and shifts away from fossil fuels to low-carbon energy sources
Buildings sector CO2 emissions by subsector in the CTS
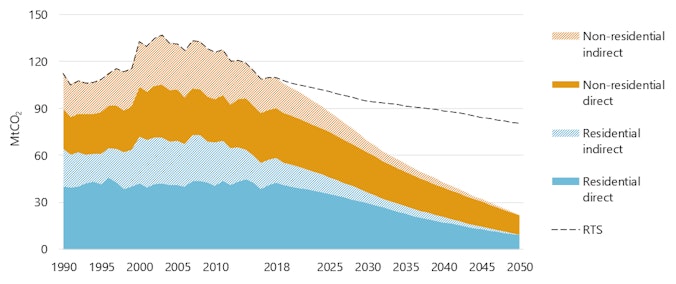
IEA 2019. All rights reserved.
Building technology improvements are critical to reduce energy demand
The energy intensity of space heating in residential buildings in Canada today ranges on average between 75 kilowatt-hours per square metre (kWh/m2) for newer construction to as much as 220 kWh/m2 or more for buildings erected before 1960. The residential sector accounts for around three quarters of floor area and 60% of heating and cooling energy consumption in Canada’s buildings, and around 950 million m2 in new residential floor space is projected to be added in the CTS by 2050. To offset this growth and subsequent increase in energy service demand, high-performance nZEBs (or zero-energy-ready buildings) become the market standard by then, while all other construction moves to today’s best practice.
Deep energy renovation of existing building stock is equally central to reduce heating and cooling energy demand in buildings. Around 60% of current residential floor area undergoes a major energy renovation by 2050 in the CTS, bringing the average heating intensity of those renovated buildings down to about 100 kWh/m2. Around half of existing non-residential floor area is also renovated, reducing the average heating intensity of those buildings by around 40%-50% in most provinces by 2050. Altogether, including high-performance new construction, the result is that the average space heating intensity of Canada’s buildings stock by 2050 is a third lower than in 2018.
High-performance new construction and deep energy renovations of existing buildings could lower the average space heating intensity of Canada’s buildings by a third by 2050
Evolution of building stock heating intensity by vintage
Achieving CTS ambitions for building envelope improvements will depend on a number of factors, including the type of building, its location and the cost-effectiveness of the applied technologies. Multiple building envelope measures with a relatively short payback period (e.g. 10-15 years) already exist today, while others require possible incentives or policy push to address upfront investment costs or longer returns on investment. Deep energy renovations naturally drive up costs and the payback period, although some of this is due to low demand for those measures today.
Energy prices play a strong role in the attractiveness of building envelope improvements, especially in provinces where natural gas prices are low. For example, gas prices in Ontario are around USD 25 per megawatt-hour (MWh), which means investment in a typical multifamily apartment building may make economic sense only for measures that achieve around 20% energy intensity improvement (assuming a 15-year payback period). In Alberta, where natural gas prices are about USD 15/MWh, cost-effective building envelope measures may achieve only 10-15% energy intensity improvement or less. By contrast, improvements of 30% or more may be possible for buildings using electricity for space heating, even in provinces with low electricity prices, such as Quebec.
Energy prices in some regions will play a strong role in the economic attractiveness of deep energy renovations of existing buildings and may require additional policy signals
Example range of cost-effective building energy renovations relative to payback period
IEA 2019. All rights reserved
Energy prices equally affect the economic attractiveness of clean energy technology for heating in buildings. For example, switching from natural gas furnaces to electric heat pumps in provinces with low gas prices such as Alberta or Saskatchewan may be cost-effective for only a small number of buildings (e.g. in new construction). In others, such as Ontario and Quebec, as many as 5 million heat pumps and efficient biomass stoves could be deployed cost-effectively in the CTS under projected energy prices. In British Columbia, where electricity prices and milder winters favour heat pumps, the vast majority of heat pumps recommended in the CTS could be implemented cost-effectively today.
Policy signals and other market incentives will most likely be needed to drive investment in building technology solutions that help realise the clean energy transition
Cost-effective measures relative to energy prices and CTS recommendations
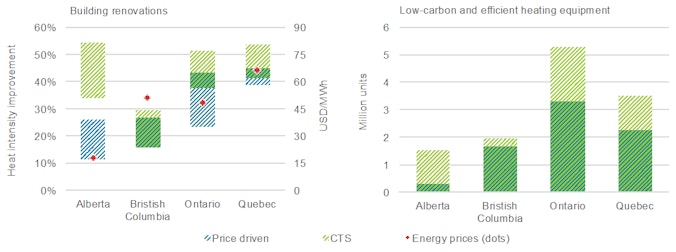
Notes: The blue-hatched area represents cost-effective measures under current techno-economic conditions. The overlap of blue and green hatched areas represents CTS measures that are cost-effective. The green-hatched area shows CTS measures that would require policy signals or economic incentives to be realised. For all assessments, an annual discount rate of 2.5% was used. IEA 2019. All rights reserved.
Assertive policy signals are needed to achieve clean energy ambitions
Techno-economic considerations alone would not achieve ambitions set forth in the CTS and only achieve around half of the energy demand reductions needed to put Canada’s buildings sector on a sustainable pathway. Economic conditions themselves also do not typically explain decision making around clean energy technology in the buildings sector – for example, many buildings still do not undergo major energy renovations, even when they are cost-effective. While not infeasible, phasing out natural gas use in buildings is also unlikely, because of embedded infrastructure (especially in dense urban areas with large shares of multifamily buildings) and also given Canada’s abundant natural gas resources.
Achieving major improvements in building energy performance and shifts in heating and cooling equipment choice (e.g. to replace gas-fired technology with other alternatives) will require ambitious policy. A transparent and long-term policy vision is needed to provide industry, investors and other market actors with the necessary signals to make informed decisions. For example, clear strategy and guidance on future natural gas use in buildings could outline limitations on future network expansion and define progressively tighter energy performance requirements for gas-based equipment. These signals would help avoid potential lock-in of energy demand or eventual expensive changes to buildings-related assets.
Additional policy signals, building upon Canada’s extensive experience with energy performance standards and building energy codes, are needed to deliver on the energy savings potential and related emissions reduction highlighted in the CTS. Energy policy can build upon multiple possible levers to enable the transition to sustainable heating and cooling in buildings. For example, market-based solutions such as on-bill financing and energy service contracts can address well-known issues in the buildings sector, such as lack of private investment despite cost-effectiveness of measures. Collaboration with industry can also help ensure the right scope and scale of potential building energy performance measures, improving the market offer and the overall affordability of clean energy technology. Ideally, these would be paired with pricing tools such as Canada’s current carbon tax and expansion of energy performance standards, for instance setting progressively higher minimum energy performance requirements for existing buildings
Considering cost-effective measures relative to projected energy prices may not be sufficient on its own to deliver on ambitions for the clean energy transition in some regions
Space heating reductions from effective measures relative to energy prices
These policy measures, alongside continued support for technology improvements and product innovation, would help address current technical or economic gaps in the market. For example, the average operating cost to run an electric heat pump across Canada’s provinces and territories is only marginally lower than operating a condensing gas boiler, given the spread of natural gas and electricity prices relative to typical equipment performance. In some provinces, shifts to heat pumps may not have any positive return on investment (from a purely economic perspective). This suggests government policy has a critical role in shaping market demand: first and foremost through minimum energy performance standards, which have a strong track record of paying for themselves when done in consultation with industry and when using appropriate market incentives. Further innovation – for example, working with manufacturers to improve heat pump performance in cold climate conditions – will also help narrow the spread of operational costs, making those products more attractive.
In some markets, for example in Quebec and Manitoba where electricity prices tend to be lower, policy can play a similar role in guiding market decisions, even in conditions that from an economic perspective are already favourable. This includes use of market incentives such as rebates or utility reward programmes to drive technology demand as well as use of labelling, certification programmes and other information tools that help steer demand towards clean energy technologies.
Broadening economic assessments to include other benefits beyond simple return on investment can also help drive clean energy technology demand. Energy efficiency measures in buildings lead to electricity savings that can support lower investment needs to build, operate and maintain power generation and network capacity. Healthy, well-ventilated and insulated buildings generally last longer, often have higher property values and typically increase productivity, all of which could be integrated in the way building energy performance measures are assessed. To do this, market frameworks need to enable investors and related stakeholders to integrate these elements in their decision making – for instance, working with insurance companies and mortgage lenders to reflect the value added from sustainable buildings when assessing financial risks.
Policy support is needed to encourage shifts to efficient heat pumps in regions where natural gas and electricity prices mean there may be little economic incentive to change equipment
Space heating operating cost, 2018
Heating in Canada could look very different by 2050
Canada is a large and diverse country, and energy is produced and consumed differently across its provinces and territories. Energy resources, historical infrastructure, energy policy and environmental regulations all influence energy decisions in the buildings sector, together with consumer preferences and climatic conditions. For example, Atlantic Canada and the territories use a much higher share of refined products than the rest of the country, primarily due to the limited availability, infrastructure limitations and relatively higher cost of other energy sources. These regions notably rely on liquid fuels for heating, for economic reasons and because it can be transported by truck.
In the CTS, the mix of fuel and energy technology for heating in buildings looks considerably different from today, although it still takes into account distinctions across Canada’s provinces. In provinces with large hydroelectric infrastructure such as Quebec, electricity continues to dominate heating energy use in buildings, although electric resistance heating equipment is progressively replaced with much more efficient electric heat pumps. Use of oil boilers and gas furnaces is dramatically reduced, while modern biomass, especially given Canada’s considerable bio-resources, represents a rising share of heating in Quebec, Atlantic Canada and the territories.
By contrast, much of western Canada and Ontario are likely to continue using natural gas, given the well-developed gas pipelines and projected low prices. Steady shifts take place to more efficient equipment such as gas thermal and hybrid heat pumps, while electric heat pumps grow in some building types, particularly in detached single-family dwellings and in new construction. Use of solar thermal energy also increases, particularly in high-performance nZEBs and zero-energy-ready buildings, although the share of solar in total heating energy consumption remains relatively small.
Overall, nearly 45% of Canada’s heating equipment stock in 2050 in the CTS is high-performance electric heat pumps, whose average seasonal efficiency increases from around 2.5-3.0 today (compared with most gas condensing boilers with efficiencies of 0.92-0.95) to between 3.5 and 5.0 by the 2030s, thanks to support for technology innovation and greater market demand. The share of fossil fuel used for heating in buildings (mostly natural gas) drops to around 30% by 2050, while oil-fired boilers, which represent around 13 % of Canada’s heating demand today, are almost phased out by 2050.
Clean energy technology progressively displaces use of fossil fuels for heating in buildings in the CTS
Share of heating energy consumption in the CTS by fuel and region
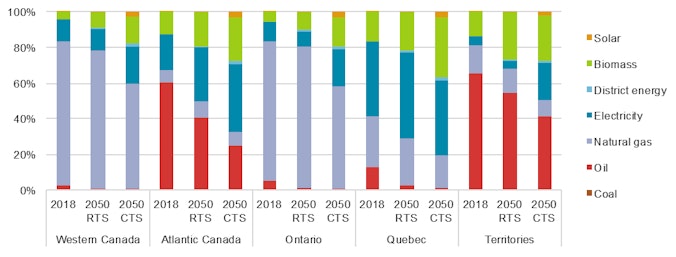
IEA 2019. All rights reserved.
Support for innovation can identify appropriate market solutions
The CTS considers existing energy technology solutions that can achieve sustainable heating and cooling demand in buildings in Canada, including some technology performance improvements and eventual cost reductions that are achieved through market economies of scale. Those improvements would require effective policy signals as well as support for product innovation that delivers affordable, efficient and low-carbon heating and cooling solutions for buildings.
One such example is continued support for heat pumping technologies in cold climate conditions. Heat pump performance today (for air-source heat units) typically drops at outdoor temperatures below 0-5°C, and even cold-climate heat pumps can struggle in temperatures below -10°C. To address these types of technical issues, heat pumps are often oversized to ensure proper heat delivery on extremely cold days, or they can be paired with other equipment, such as electric resistance heaters or backup gas furnaces. Yet actual temperature profiles across Canada’s provinces suggest that the number of very cold and extremely cold days represents less than 10% of total winter heat demand.
This suggests there is opportunity to continue research and development programmes that identify appropriate technology solutions to ensure high-performance equipment that is responsive to heating needs in all conditions. Potential solutions already being considered in Canada through research being led by Natural Resources Canada include hybrid (e.g. natural gas and electric) heat pump solutions that would ensure heat production at very cold temperatures while equally achieving very high energy performance levels at typical winter temperatures. Other technical considerations include design of dual compressor units that meet heating demand on extremely cold days (at full load) while maximising energy performance at low partial loads during warmer winter days.
New technology solutions such as hybrid heat pumps could help increase the overall energy efficiency of heating equipment, guaranteeing performance even on the coldest of winter days
Heat demand distribution by province relative to outside temperature, 2018
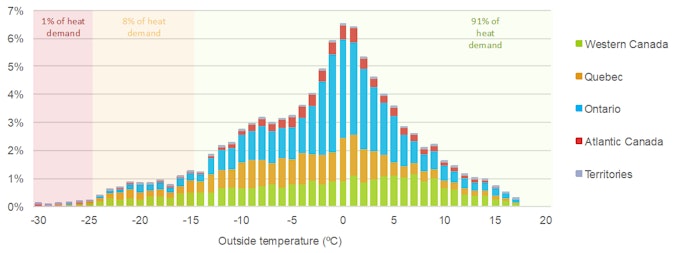
Source: Derived with data on hybrid heat pump performance in Canada from the research centre in Ottawa of Natural Resources Canada.
These technology considerations equally apply to air-conditioning technologies for summertime cooling demand. A considerable number of buildings in Canada use air conditioning in the summer, particularly in Quebec and Ontario, where hot and humid summer weather can lead to felt temperatures of 35°C or more. In fact, while the average number of cooling degree days (CDDs) in Canada is quite low compared with other countries with high air conditioner (AC) ownership, high levels of humidity mean the vast majority of those of those CDDs are at relative humidity higher than 50%.
Technology innovation – for example, use of solid or liquid desiccants to treat latent (humid) heat – could improve the energy performance of air-conditioning equipment in buildings while ensuring thermal comfort. The Canadian government can work with industry and international partners to identify and deploy innovative technology solutions that meet rapidly growing cooling demand in different climate contexts. This includes support of research collaboration programmes, such as work being led by the IEA Technology Collaboration Programmes (TCPs) on Heat Pumping Technologies (HPT TCP) and Energy Storage (ECES TCP) to deliver an affordable, energy-efficient and low-carbon “Comfort and Climate Box”.
Technology innovation to address operational issues such as treatment of latent heat can improve the energy performance of air-conditioning equipment and overall thermal comfort in buildings
AC ownership relative to CDDs and humidity, 2018-50
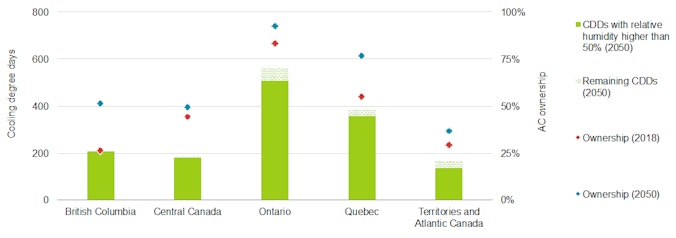
IEA 2019. All rights reserved.
The transition to sustainable buildings can come at a lower cost
Capital spending for heating, cooling and building envelope investments in the CTS increases by around 5% in the coming decade, relative to expected trends to 2030 in the RTS. This corresponds to an extra USD 3-4 billion in additional annual spending in order to deploy low-carbon and energy-efficient technology solutions for buildings. Yet total spending on buildings would actually be lower than the RTS in the 2030s and beyond, with annual savings reaching as much as USD 20 billion in 2050.
These long-term savings are achieved through improved economies of scale from widespread deployment of clean energy technologies, which are supported by assertive policy measures and greater support for research and development that helps to deliver technology solutions at affordable prices. Investment in deep energy renovation of existing buildings also leads to substantial savings in capital expenditure over time, as these types of renovations typically extend the lifetime of a building, thereby reducing subsequent need for new construction.
Capital improvements lead to major reduction in energy demand for space heating and cooling in buildings, which subsequently leads to important reductions in energy spending for households and businesses. In fact, overall spending on energy for heating and cooling in buildings decreases by nearly 15% compared with today, despite projected increases in electricity prices (ranging between 20% to 35% higher than today by 2050 across Canada’s provinces and territories) and the strategic shift from cheap natural gas to more expensive electricity.
Upfront investment in clean energy technologies in the CTS leads to savings in capital and operational expenditures for heating and cooling in buildings as early as the 2030s and beyond
Heating and cooling expenditures in the CTS relative to the RTS, 2018-50
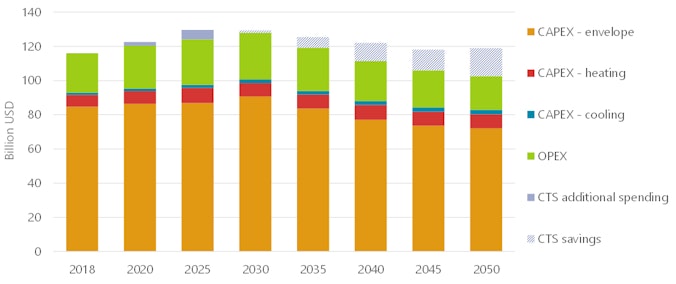
Notes: CAPEX = capital expenditure. OPEX = operational expenditure. IEA 2019. All rights reserved.
Lessons for heating and cooling strategies from Canada’s provinces
The CTS highlights a pathway towards low-carbon and energy-efficient heating and cooling in buildings for Canada’s provinces and territories, all of which have the potential to ensure thermal comfort while equally reducing reliance on fossil fuels and buildings-related CO2 emissions. Yet current and projected energy prices – and in particular low natural gas prices – pose a particular challenge in most Canadian regions, as they do in many other countries. Market appetite for clean energy technology also often does not reflect what is technically and economically feasible, underscoring the complex nature of the buildings sector and the multiple factors influencing its actors and decision makers.
The good news is that the toolkit needed to realise the potential highlighted in the CTS includes a suite of policies, technologies and financing tools with proven track records. Government policy can shape market demand first by setting clear ambitions for sustainable heating and cooling in buildings. This includes committing to specific targets on building energy performance and carbon intensity in order to send long-term signals to market actors and address risks associated with clean energy investment – for instance in markets such as Quebec where current energy prices already favour building performance improvements.
Canada can also build upon its long-standing experience with minimum energy performance standards, which have a strong record of paying for themselves when done in consultation with industry and when using appropriate market incentives. This includes ratcheting up current requirements to steer market shifts towards clean energy technologies such as electric, gas thermal and hybrid heat pumps, even in places with low natural gas prices such as Ontario and Alberta. Benchmarking across technologies (e.g. common performance standards for gas furnaces and heat pumps) would also help to encourage greater competition and technology innovation.
Support for innovation can equally make sure clean energy technologies are fit for purpose in all markets and conditions, addressing issues such as higher upfront prices, performance in challenging conditions such as extremely cold winter days in Manitoba, and lack of adaptability to certain building contexts (e.g. multifamily residential buildings with limited outdoor space for exterior heat pump units).
Policy can also help guide market decisions, even in conditions that from an economic perspective are already favourable, like in British Columbia. This includes use of market incentives such as rebates or utility reward programmes to drive technology demand and establish appropriate economies of scale. Use of labelling, certification programmes and other information tools can help steer demand towards clean energy technologies.
Building energy renovations play a central role in the CTS, and improving the energy performance of existing buildings will require greater use of financial tools, market mechanisms and innovative business models to accelerate the transition. The Canadian government can enable this through policy intervention that shapes market rules to improve access to finance, de-risk clean energy investment and broaden availability of market-based instruments that lower the barriers for a clean energy transition. Greater effort also needs to be focused on compiling a comprehensive and sound evidence base of technical and economic solutions, including pilot programmes such as two pilot “Energiesprong” projects in Ontario and British Columbia. In parallel, provinces and territories can introduce regulatory normative measures such as building energy labelling schemes and codes for renovations that set either minimum technical requirements or minimum energy performance levels.
The breadth of energy prices, technology choices, building types and climatic conditions in Canada illustrates that sustainable heating and cooling will require comprehensive policy packages that bring together multiple technical and market-based solutions under one umbrella. An across-the-board framework that delivers cost-effective action tailored to specific building needs using the most effective technology solutions is possible, but it will require a more ambitious regulatory framework. Collaboration with industry and international partners can help achieve this, sharing knowledge and best practices, increasing access to finance, and developing and deploying clean energy technologies that deliver affordable, energy-efficient and low-carbon heating and cooling in buildings.